
These methods also suffer from low spatial and/or temporal resolution, as well as the need for large patient cohorts and consortia to identify the features of the human brain connectome related to normal aging or disease. Moreover, only a limited number of scientists and clinicians master the necessary expertise in, or have access to, brain imaging techniques such as non-invasive functional magnetic resonance imaging (fMRI) and electroencephalography (EEG), or minimally invasive methods such as the implantation of recording and stimulating electrodes in the brain. Unlike rodent models, access to living human test subjects and primates, or ex vivo brain slices derived thereof, is surrounded with ethical issues. In light of this broad spectrum of species-specific differences on the level of DNA, molecular pathways, cells and brain networks, it should not come as a surprise that the modeling in rodents of complex neurodegenerative diseases and psychiatric disorders pose challenges, in particular for drug and target discovery. Ultimately, they may lead to changes in interactome at the mesoscale, such as lipid profiles or the composition of the synaptic proteome. Species-specific gene expression patterns are found in the context of neural circuit function and specific cell-types, and they may be related to the organization of cis-regulatory elements in DNA or stress conditions such as aging. Evolution also had its impact on the divergence of genetic make-up of humans compared to other mammals, notably the expression of non-coding RNAs.
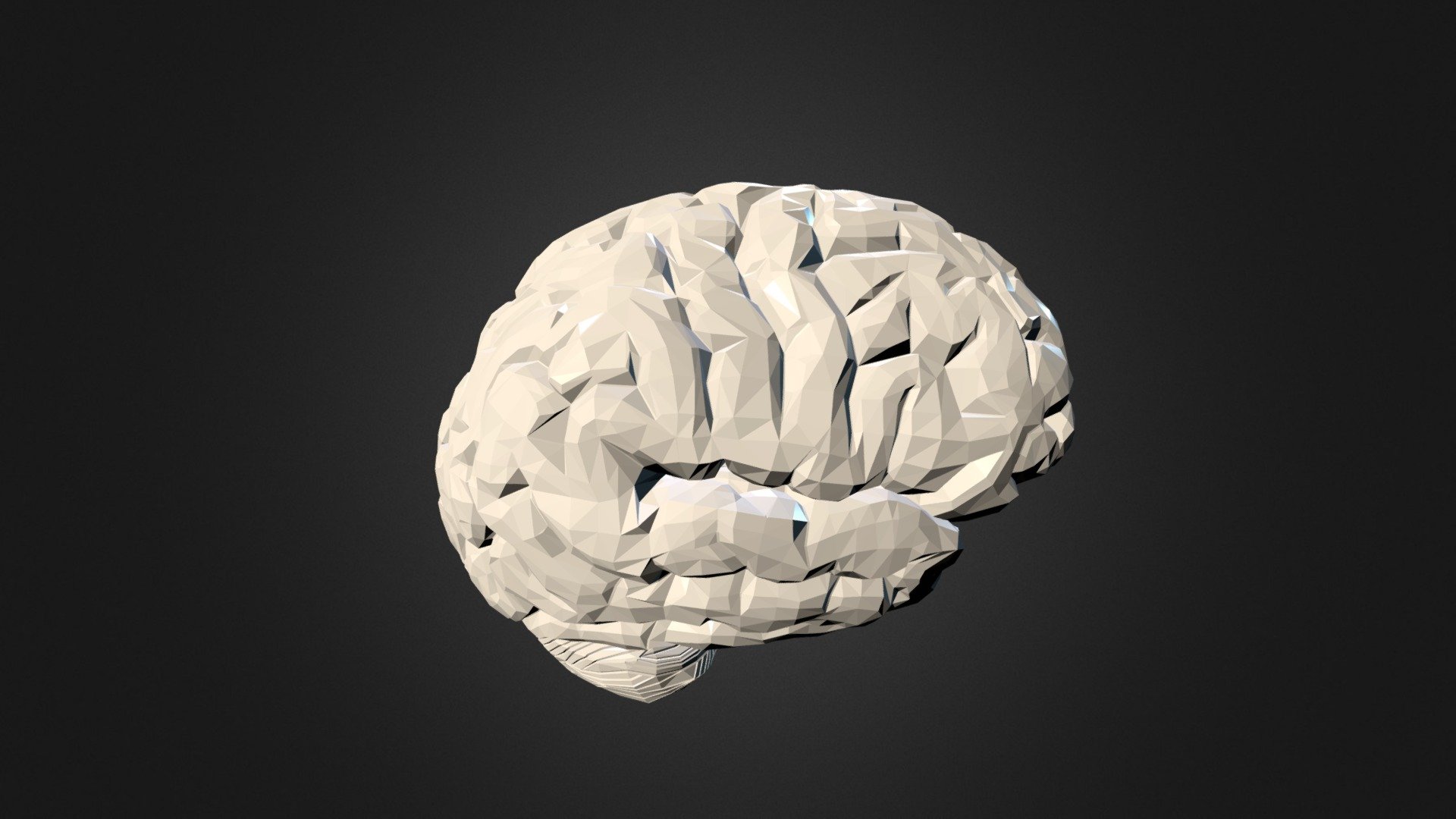
The cerebral cortex, the part of the brain involved in motor and sensory function and processing information, as well as associated with psychiatric diseases, is less complex in rodents in comparison to primates in general, and to humans in particular. However, these model systems do not fully recapitulate the complexity of the human brain related to neural circuit architecture, genetics, gene expression, cell-type diversification, lipidomics and proteomics. Animal models, such as rodents, primates, worms and experimental systems such as yeast, have yielded great insights into development, function and diseases of the brain. To understand human brain physiology, the human condition must be studied in a laboratory setting. Perspectives on designing improved tissue models of the human brain are offered, focusing on an integrative approach merging biomedical engineering tools with organoid biology. The contributions of in vitro 3D tissue culture systems to new insights in neurophysiology, neurological diseases and regenerative medicine are highlighted. This review article describes recent technological advances in engineering 3D cultures that more closely resemble the human brain.

The second approach is organoid technology, in which stem cells are exposed to chemical and/or biological cues to activate differentiation programs that are reminiscent of human (prenatal) development. The first is biomedical engineering methodology, including bioengineered materials, bioprinting, microfluidics and bioreactors, used alone or in combination, to mimic the microenvironments of native tissues. Two parallel approaches have emerged for the design of 3D culture systems. The discrepancy between in vivo and in vitro tissue-cell-molecular responses impedes understanding of human physiology in general and creates roadblocks for the discovery of therapeutic solutions.

3-dimensional (3D) laboratory tissue cultures have emerged as an alternative to traditional 2-dimensional (2D) culture systems that do not recapitulate native cell behavior.
